Abstract: Using biobased microcrystalline cellulose (MCC) as the raw material, MCC was coated and modified by gradually adding melamine (MEL) and phytic acid (PA) to prepare a biobased hybrid structure of phytate amine-coated microcrystalline cellulose (MEL-PA-MCC). The structure, elemental composition, and morphology of MEL-PA-MCC were characterized using Fourier transform infrared spectroscopy, thermogravimetric analysis, differential scanning calorimetry, scanning electron microscopy, X-ray photoelectron spectroscopy, energy dispersive spectrometry, and X-ray diffraction. The results showed that after modification with MEL-PA, MCC exhibited characteristic absorption peaks of C=N, P=O, and P—O at 1 624, 1 328, and 785 cm-1, respectively, and the maximum thermal decomposition rate significantly decreased, while the char yield increased; the surface of the MEL-PA-MCC hybrid structure was densely attached with round particles, and the N and P elements were significantly enhanced, and the crystallization behavior was also significantly changed. These results collectively indicate that the phytate amine successfully achieved the coating and modification of MCC. When MEL-PA-MCC was used to modify poly(butylene adipate-co-terephthalate) (PBAT), the composite material could achieve a UL-94 V-1 rating in vertical burning tests when the mass fraction of MEL-PA-MCC added was 5%, and the limiting oxygen index increased to 25.5%, with significant improvement in anti-dripping performance. In addition, the tensile strength and tensile elastic modulus of the PBAT/5%MEL-PA-MCC composite material were increased by 87.31% and 41.30%, respectively, compared to pure PBAT. Therefore, the introduction of the prepared MEL-PA-MCC flame-retardant hybrid structure can significantly improve the flame retardancy and tensile properties of PBAT.
keywords: phytic acid; melamine; microcrystalline cellulose; flame retardant; hybrid structure
Microcrystalline cellulose (MCC) is a polysaccharide substance connected by β-1,4-glycosidic bonds, with a crystallinity generally ranging from 55% to 80% [1-2]. MCC is widely used in fields such as food, medicine, and packaging due to its biodegradability, low cost, and wide availability [3-6], exhibiting high scientific research value. Additionally, MCC has a carbonization effect at high temperatures, forming a carbonized layer that prevents the diffusion of combustion products into the material [11]. MCC shows high thermal stability, thus possessing excellent application potential in flame-retardant polymers. Therefore, by conducting flame-retardant modifications on MCC, constructing hybrid structures rich in flame-retardant elements, and applying them in polymer matrices, it has gradually attracted attention in the field of flame retardancy. Currently, common MCC modifications mainly include acid modification [12], etherification [13], esterification [14], oxidation [15], and ionic liquid modification [16]. Introducing other elements through chemical modification methods on the surface of MCC to prepare biomass hybrid flame retardants has become one of the current research hotspots.
In recent years, the preparation of hybrid flame retardants by introducing organic or inorganic particles rich in P, N flame-retardant elements for modifying composite materials has received considerable attention. Phytic acid (PA) is a phosphoric compound with high phosphorus content, featuring good biocompatibility, environmental friendliness, and certain flame-retardant properties. Under high-temperature conditions, PA decomposes to produce phosphoric acid and water, where phosphoric acid plays a role in reducing the flammability of materials [17-19]. For instance, Xiao Wencheng [18] used chitosan hydrogel as a carrier, forming a three-dimensional network structure with PA, and doped it with nanoscale silica sol to prepare flame-retardant polyester fabric. The results showed that the treated fabric had better thermal stability, with an increase of 41.5% in char yield, no melt dripping, achieving a synergistic flame-retarding effect among phosphorus, nitrogen, and silicon. Additionally, melamine (MEL), due to its high nitrogen content, low cost, and easy availability, can serve as a gas source in intumescent flame retardants, releasing a large amount of inert gases during combustion, playing an excellent synergistic flame-retarding role in the gas phase [20]. He Wenping [21] synthesized diphenylamine phosphate melamine salt (DPMS) through further reaction with MEL using o-phenylenediamine and phosphoryl trichloride as raw materials, and blended it with nylon 6 by melting. The results indicated that DPMS could significantly improve the flame-retardant performance of the nylon 6 composite, passing the UL-94 test at V-0 level. Luo Minyi [22] prepared polyphosphate (MPP) mainly from MEL and phosphoric acid, adding it to polyvinyl alcohol (PVA). When 16.6 wt% MPP was added, the PVA composite material reached a flame-retardant grade of V-0, with a significant reduction in heat release rate and a noticeable improvement in mechanical properties.
Introducing PA and MEL into the carrier, a MEL-PA hybrid flame retardant structure was prepared for modifying the polymer matrix. It is reported that Li Wenxiong [23] further reacted the prepared MEL-PA with transition metal ions Mn2+, Zn2+, Ni2+ to prepare a bio-based hybrid flame retardant for flame-retardant polypropylene. Zhang Bing et al. [24] prepared MEL-PA/RPUF composites by melting and mixing self-made MEL-PA with rigid polyurethane foam (RPUF) at a certain ratio. The study showed that compared with pure RPUF, the thermal stability of MEL-PA/RPUF composites was higher, and the residual carbon rate significantly increased to 20.6%. Wang Yiwen et al. [25] blended MEL-PA with nylon 66 by melting to prepare composites. Test results indicated that MEL-PA improved the flame retardancy of nylon 66. When the mass fraction of MEL-PA reached 8%, the UL-94 test rating of the composite could reach V-0 level, the limiting oxygen index (LOI) was greater than 27%, and the total smoke release was significantly reduced. Although both PA and MEL as raw materials for flame retardant modification have good flame retardant effects, there are few reports on the use of PA and MEL to coat and modify MCC to prepare hybrid flame retardants.
Therefore, the author uses MCC as a carrier and adopts PA and MEL to encapsulate and modify MCC, preparing phytic acid amine encapsulated MCC (MEL-PA-MCC) bio-based hybrid flame retardant material. The influence of different molar ratios of PA and MEL on the structure and composition of MCC is explored. The structure, morphology, elemental composition, and thermal stability of MEL-PA-MCC are systematically characterized. MEL-PA-MCC is applied to a biodegradable matrix - poly(butylene adipate-co-terephthalate) (PBAT), and the flame retardancy and tensile properties of PBAT/MEL-PA-MCC composites are analyzed.
1 Experimental Section
MCC: column chromatography, China National Pharmaceutical Group Chemical Reagents Co., Ltd.
PA: Purity 70%, Shanghai Macklin Biochemical Technology Co., Ltd.;
MEL: 99% purity, Shanghai Macklin Biochemical Technology Co., Ltd.;
Formaldehyde: purity 37%, Shanghai Macklin Biochemical Technology Co., Ltd.;
PBAT: 1908, injection molding grade, Shanxi Jinhui Zhaolong High-tech Co., Ltd.
1.2 Main Instruments and Equipment
Fourier Transform Infrared (FTIR) Spectrometer: Nicolet IS50, Themo Fisher, USA;
Thermogravimetric (TG) Analyzer: 219 F3, Netzsch Instruments, Germany;
Differential Scanning Calorimetry (DSC) Analyzer: DSC 214, Netzsch Instruments, Germany;
Scanning Electron Microscope (SEM): Quanta 250 FEG, FEI Company, USA;
X-ray photoelectron spectroscopy (XPS) instrument: ESCALAB QXi X, Thermo Fisher Scientific, USA;
Energy dispersive spectrometer (EDS): INCA-350, Oxford Instruments, UK;
X-ray diffraction (XRD) instrument: XRD-6100, Shimadzu Corporation, Japan;
Torque rheometer: HAAKE Polylab OS, Haake Technik GmbH, Germany;
Electric vulcanizing molding machine: ZHY-W, Hebei Chengde Testing Machine Co., Ltd.;
LOI instrument: JF-3, Nanjing Jiangning Analytical Instrument Factory;
Horizontal and Vertical Burning Tester: CZF-3, Nanjing Jiangning Analytical Instrument Factory;
Universal Testing Machine: INSTRON3365, Instron (Shanghai) Testing Equipment Trading Co., Ltd.
1.3 Preparation of MEL-PA-MCC
Study the effect of coating modification of MCC with different amounts of phytamine. First, 3 mL of 13.5 mol/L formaldehyde solution and a certain amount of MEL were added to 150 mL of deionized water, and the mixture was rapidly stirred at 80 ℃. After the solution became clear, 5 g of MCC and 5 mL of 13.5 mol/L formaldehyde solution were added. Based on the chemical reaction formula shown in Figure 1, the molar ratio of PA to MEL is 1:6. A certain amount of 1.5 mol/L PA solution was slowly added and rapidly stirred, and the reaction was carried out at 80 ℃ for 5 h. The detailed formulation is shown in Table 1. Finally, the precipitated product was collected by vacuum filtration, washed three times with deionized water at 80 ℃ until the pH of the washing filtrate reached 7. The washed product was then vacuum dried at 100 ℃ for 12 h to obtain MEL-PA-MCC powder, which was then ground and packed.
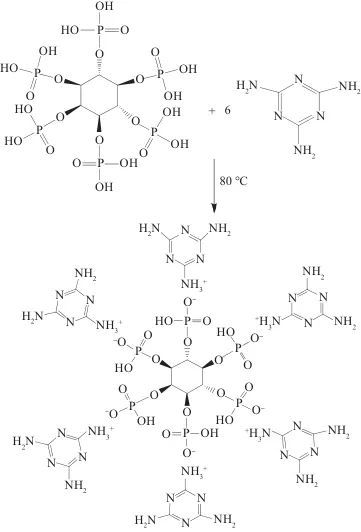
Fig. 1 Reaction formula of phytamine
Tab. 1 Formulation of MEL-PA-MCC ( g )
1.4 Preparation of PBAT/MEL-PA-MCC Composite Materials
PBAT pellets and MEL-PA-MCC flame retardant were vacuum dried at 85 ℃ for 12 h before use. The PBAT/MEL-PA-MCC composite was prepared by melt blending for 8 min in a torque rheometer at 160 ℃ and 60 r/min. The specific formulation composition is listed in Table 2. The mixed samples were placed into molds and hot pressed in an electric vulcanizing machine, with a hot pressing temperature of 170 ℃, hot pressing time of 15 min, and venting 10 times, followed by cold pressing for 8 min at 20 ℃. According to the requirements of the UL-94 vertical burning test, LOI, and tensile property tests, the samples were cut into standard size strips for flame retardancy and tensile property testing.
Table 2 Composition ratios of different PBAT/MEL-PA-MCC composites
1.5 Testing and Characterization
FTIR analysis: the scanning range is 400~4,000 cm-1, with a resolution of 4 cm-1, and the FTIR spectrum is obtained after 16 scans.
TG analysis: The sample weight is around 5 mg, loaded in an Al2O3 crucible, under N2 atmosphere, with a heating rate of 10 K/min from 50 ℃ to 800 ℃, the purge gas flow rate is set to 40 mL/min, and the protective gas flow rate is set to 60 mL/min. Record the TG and first derivative curves of the sample.
DSC analysis: The sample weight is about 5 mg, loaded in an aluminum crucible, under N2 atmosphere, heated from 20 ℃ to 250 ℃ at a heating rate of 10 K/min, with the purge gas flow set at 40 mL/min and the protective gas flow set at 60 mL/min. The DSC curve of the sample is recorded.
XRD characterization: operating voltage of 60 kV, current of 40 mA, scanning step width of 0.02°, scanning range diffraction angle 2θ from 0° to 80°.
XPS testing: Scanning the surface of pure MCC and MEL-PA-MCC to determine the elemental composition and content, and further identify the valence bond structure of the elements contained.
SEM observation: After drying and grinding the sample, it was evenly spread on the conductive adhesive, and then subjected to surface gold sputtering treatment. The micro-morphology of pure MCC and its modified form was observed under a scanning voltage of 10 kV.
EDS testing: Analyze the elemental content of pure MCC and MEL-PA-MCC at the same acceleration voltage.
Flame retardancy test: A vertical burning tester is used, and the sample is tested for vertical burning according to GB/T 2408-2021; an LOI tester is used, and the sample's LOI value is determined according to GB/T 2406.3-2022.
Tensile performance test: Using a universal testing machine, the samples are subjected to tensile testing according to GB/T 1040.1-2018, with a tensile rate of 50 mm/min. At least five samples from each component are measured, and the results are averaged, with a standard deviation within 5%.
The FTIR spectra of pure MCC and MEL-PA-MCC are shown in Figure 2. The pure MCC sample has a stretching vibration peak of O—H bond at 3,340 cm-1, a broad peak of C—H bond stretching vibration at 2,900 cm-1, bending vibration peaks of O—H bond and C—H bond at 1,653 cm-1 and 1,428 cm-1 respectively, stretching vibration peaks of C—O—C and C—C bonds at 1,160 cm-1 and 1,107 cm-1, a vibration peak of C—O bond at 1,030 cm-1, and an out-of-plane bending vibration peak of C—H at 890 cm-1. In addition to the same peaks as MCC, MEL-PA-MCC also exhibits distinct characteristic absorption peaks, such as C=N peak and —NH deformation vibration peak in the MEL triazine ring at 1,624 cm-1 and 1,526 cm-1, and a C—N bond stretching vibration peak at 812 cm-1. Moreover, characteristic peaks of P=O and P—O in the PA structure are observed at 1,328 cm-1 and 785 cm-1, with 936 cm-1 representing the C—H group absorption peak. These results indicate that the corresponding functional group absorption peaks of MEL-PA-MCC composites were detected, and there was no significant difference in the FTIR spectra of each MEL-PA-MCC with the increase in PA and MEL content. Therefore, it can be preliminarily judged that phytic acid amine achieves the coating modification of MCC.
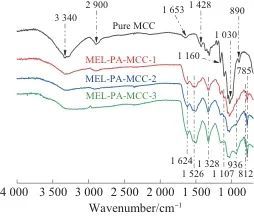
Fig. 2 FTIR spectrum of pure MCC and MEL-PA-MCC
2.2 Thermal Stability Analysis
Figure 3 shows the TG and DTG curves of pure MCC and MEL-PA-MCC, with the corresponding data listed in Table 3. The initial decomposition temperature of pure MCC is 315.9 ℃, and the char yield is 2.78%. However, the TG data for MEL-PA-MCC show significant changes; compared to pure MCC, although the initial decomposition temperature of MEL-PA-MCC decreases, the decomposition rate significantly slows down, and the char yield greatly increases. For example, the initial decomposition temperature of MEL-PA-MCC-3 is 230.7 ℃, with a maximum thermal decomposition rate of only 2.92%/min, and the char yield can reach 26.51%. Based on literature review [26-27], it is preliminarily determined that the first degradation stage of MEL-PA-MCC is due to PA decomposing to produce phosphoric acid, which forms phosphates with free radical groups on the surface of the material [28]. The decomposition of PA releases a large amount of heat, so the weight loss rate at this stage is relatively high; the second stage involves the decomposition of MEL to produce nitrogen and ammonia, etc. [29]; the third stage includes carbonization reactions from the decomposition of the material, as well as the decomposition of residual phosphate substances and inorganic salts [30]. From this, it can be seen that in MEL-PA-MCC, the phosphoric acid groups of PA decompose at lower temperatures to form a protective layer, maintaining good initial thermal stability, while the later stages of degradation see a significant increase in the catalytic charring of modified MCC, leading to an increased char yield. This indicates that after being coated and modified by phytic amine, the charring ability of MCC is notably improved. The results of TG analysis can provide a reference for further research on flame-retardant modification of hybrid MCC structures.
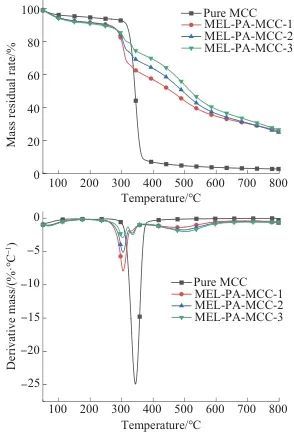
Fig. 3 TG and DTG curves of pure MCC and MEL-PA-MCC
Table 3 TG and DTG data of pure MCC and MEL-PA-MCC
Notes:T10% is temperature of 10% weight loss;Tmax is temperature of maximum mass loss rate.
Figure 4 shows the DSC curves of MCC and MEL-PA-MCC samples under nitrogen atmosphere, with the corresponding data listed in Table 4. As shown in Figure 4, both pure MCC and MEL-PA-MCC exhibit a single melting peak. The melting temperature of pure MCC is 61.84 ℃, with a melting enthalpy of 145.8 J/g. The melting peak of MEL-PA-MCC is significantly higher than that of pure MCC, and the melting enthalpy also increases markedly. However, as the amount of phytic amine further increases, the melting peak of MEL-PA-MCC slightly decreases. Among them, MEL-PA-MCC-3 has a melting temperature of 62.8 ℃ and a melting enthalpy of 205.6 J/g. Therefore, the crystallization behavior of MEL-PA-MCC has changed significantly, which is due to the coating of phytic amine on the surface of MCC.
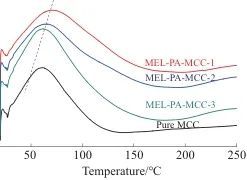
Fig. 4 DSC curves of pure MCC and MEL-PA-MCC
Table 4 DSC data of pure MCC and MEL-PA-MCC
Notes:Tm is melting temperature;ΔH is enthalpy value.
To further compare the crystal structure changes between pure MCC and MEL-PA-MCC, XRD tests were conducted to analyze the differences in crystal form structure between pure MCC and MEL-PA-MCC. The XRD patterns of pure MCC and MEL-PA-MCC are shown in Figure 5. Pure MCC shows two weak peaks at diffraction angles 2θ of 14.9° and 16.2°, corresponding to the (101) and (10
) crystal plane; the strong diffraction peak appearing at 2θ of 22.4° is the (002) crystal plane of MCC; the diffraction peak at 34.5° corresponds to the (040) crystal plane [31-32]. The diffraction peaks of MEL-PA-MCC at 2θ of 14.9° and 16.2°, originally two small sharp peaks, have become a broad peak, while the peak intensities at 2θ of 22.4° and 34.5° are significantly reduced, and the diffraction peak at 2θ=34.5° begins to gradually disappear as the amount of phytic amine added increases. Therefore, the results show that the crystallization behavior of MEL-PA-MCC has been somewhat weakened, and the crystal structure has undergone significant changes, which corresponds to the changes in crystallization behavior in the aforementioned DSC.
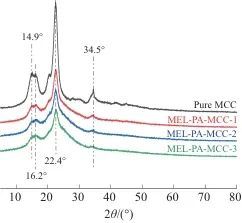
Fig. 5 XRD spectra of pure MCC and MEL-PA-MCC
To study the elemental composition and content of the products, XPS analysis was performed on MCC and MEL-PA-MCC. Figure 6 shows the XPS spectra of pure MCC and MEL-PA-MCC, with corresponding data listed in Table 5. As shown in Figure 6, the absorption peaks at binding energies of 286.81, 532.75, 399.47, and 133.57 eV correspond to C 1s, O 1s, N 1s, and P 2p, respectively. By comparison, it can be seen that pure MCC only exhibits absorption peaks for C 1s and O 1s, whereas MEL-PA-MCC, compared to MCC, has additional N 1s and P 2p, which are nitrogen from the introduced MEL and phosphorus from PA. According to Table 5, compared to pure MCC, the relative contents of C and O elements in MEL-PA-MCC-3 decreased from 58.92% and 41.08% to 46.63% and 25.91%, respectively, while the relative contents of N and P elements increased to 23.63% and 3.83%, respectively. From the above data analysis, it can be concluded that phytic acid amine was successfully coated onto MCC, consistent with the FTIR spectrum analysis.
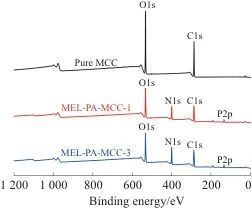
Fig. 6 XPS spectra of pure MCC and MEL-PA-MCC
Table 5 XPS test data for pure MCC and MEL-PA-MCC
2.6 Structural Morphology Analysis
The SEM morphology images of pure MCC and MEL-PA-MCC are shown in Figure 7. From Figures 7a to 7c, it can be seen that the MCC presents a neatly arranged rod-like structure with a relatively smooth surface. Under high magnification, the surface of the rod-like structure shows wrinkles or areas with a patterned texture. From the SEM images of MEL-PA-MCC-1 in Figures 7d to 7f, it can be observed that after being modified by phytic amine coating, the surface is densely covered with round particles, uniformly covering the surface of the MCC. With the increase in the amount of PA and MEL, the surface of MEL-PA-MCC-3 in Figures 7g to 7i still uniformly adheres to a large number of round particles, completely covering the MCC. The SEM images intuitively demonstrate that the phytic amine successfully achieves the coating of MCC and stably adheres to the surface of the MCC.
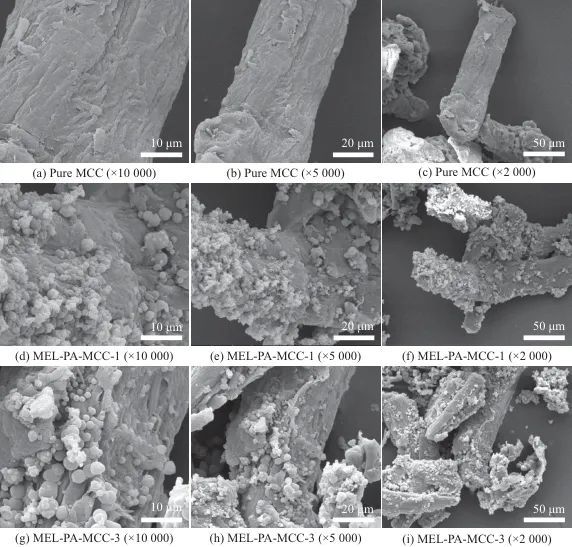
Fig. 7 SEM morphologies of pure MCC and MEL-PA-MCC
2.7 Element Composition Analysis
The EDS element distribution of pure MCC and MEL-PA-MCC is shown in Figure 8. From Figure 8, it can be seen that the distribution of each element in both pure MCC and MEL-PA-MCC is relatively uniform. Pure MCC contains two elements, C and O, with the relative content of C being 67.18% and that of O being 32.82%. In contrast, MEL-PA-MCC clearly adds two more elements, N and P, compared to pure MCC. In MEL-PA-MCC-1, the relative content of C is 57.65%, N is 13.23%, O is 26.10%, and P is 3.03%. The contents of N and P in MEL-PA-MCC-3 are further increased compared to MEL-PA-MCC-1, indicating that as the amounts of PA and MEL increase, a greater degree of coating modification of MCC is achieved. The EDS test analysis further confirms that the round particles adhering to the surface of MCC in the aforementioned SEM analysis are phytic amine, which is also consistent with the results of FTIR and XPS analyses, further verifying that phytic amine has successfully coated the surface of MCC.
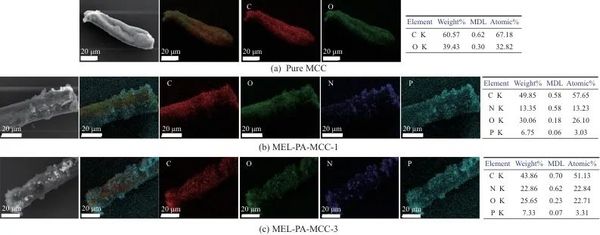
Fig. 8 EDS element diagram of pure MCC and MEL-PA-MCC
2.8 Effects of MEL-PA-MCC on the properties of PBAT/MEL-PA-MCC composites
Table 6 shows the test results of the flame retardant properties of PBAT/MEL-PA-MCC composite materials with different ratios. From Table 6, it can be seen that pure PBAT has severe dripping during combustion, no flame retardant rating, and an LOI value of 21.3%. After adding MEL-PA-MCC, the dripping phenomenon of PBAT/MEL-PA-MCC composites is significantly suppressed, the flame retardant rating gradually improves, and the LOI value increases significantly. When the mass fraction of MEL-PA-MCC added is 5% and 7%, the composites both reach V-1 level in UL-94 testing, with no dripping observed in the samples. In addition, the LOI value of the PBAT/7%MEL-PA-MCC composite material increases to 26.4%. The introduction of MEL-PA-MCC significantly improves the flame retardancy of PBAT, demonstrating excellent flame retardant effects.
Table 6 Flame Retardancy of Different PBAT/MEL-PA-MCC Composites
Note:NR refers to not reaching flame-retardant rating.
Figure 9 shows the effect of different contents of MEL-PA-MCC on the tensile properties of PBAT/MEL-PA-MCC composites. As can be seen from Figure 9, the tensile strength and tensile elastic modulus of pure PBAT are 11.43 MPa and 45.62 MPa, respectively. With the increase in the content of MEL-PA-MCC, the tensile strength of the PBAT/MEL-PA-MCC composite first increases and then decreases, but it remains higher than that of pure PBAT; at the same time, the tensile elastic modulus of the composite continuously increases with the addition of more MEL-PA-MCC. When the mass fraction of MEL-PA-MCC added is 5%, the tensile strength and tensile elastic modulus of the composite reach 21.41 MPa and 64.46 MPa, which are 87.31% and 41.30% higher than those of pure PBAT, respectively. The results show that, in addition to improving the flame retardancy of the PBAT matrix, the introduction of MEL-PA-MCC can also enhance the tensile properties of the material.
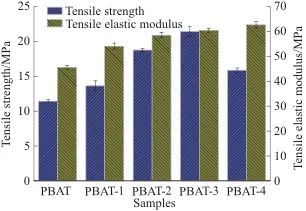
Fig. 9 Effects of different contents of MEL-PA-MCC on tensile properties of PBAT/MEL-PA-MCC composites
Using MCC as the carrier, PA and MEL were used to encapsulate and modify it, preparing a MEL-PA-MCC hybrid flame-retardant structure. The effect of adding different amounts of PA and MEL ratios on the structure, elemental composition, morphology, and thermal stability of MCC was explored. MEL-PA-MCC was introduced into PBAT resin, and its impact on the flame retardancy and tensile properties of the matrix was studied. Based on the experimental data results, the following main conclusions were obtained.
FTIR analysis indicated that MEL-PA-MCC, compared to pure MCC, mainly exhibited characteristic peaks of the triazine ring structure's C=N, N—H, P=O, and P—O at 1 624, 1 526, 1 328 cm-1, and 785 cm-1. Through SEM micro-morphology analysis, MEL-PA-MCC still had neatly arranged rod-like structures, and a large number of round particles were uniformly attached to the surface, mainly showing C, O, N, P elements uniformly adhered to the surface of MCC. The above results showed that phytic acid amine had been successfully coated on the surface of MCC.
(2) According to the thermal stability analysis, it is known that after coating modification, there is a certain degree of impact on the thermal stability of MCC. Compared with pure MCC, the maximum thermal degradation rate of MEL-PA-MCC is significantly reduced, and the char yield is significantly increased, with the highest char yield reaching 26.51%, indicating that phytic acid amine coating has significantly improved the charring ability of MCC.
(3) The crystallization behavior of phytammine-coated MCC changed significantly, with a significant reduction in diffraction peaks, indicating a decrease or weakening in crystallization behavior.
(4) The introduction of MEL-PA-MCC can effectively improve the flame retardancy and tensile properties of the PBAT matrix. When the mass fraction of MEL-PA-MCC added is 5%, the composite material reaches a flame retardant V-1 level, with LOI increased to 25.5%; compared to pure PBAT, the tensile strength and tensile elastic modulus of the PBAT/5%MEL-PA-MCC composite material are increased by 87.31% and 41.30%, respectively.
【Copyright and Disclaimer】The above information is collected and organized by PlastMatch. The copyright belongs to the original author. This article is reprinted for the purpose of providing more information, and it does not imply that PlastMatch endorses the views expressed in the article or guarantees its accuracy. If there are any errors in the source attribution or if your legitimate rights have been infringed, please contact us, and we will promptly correct or remove the content. If other media, websites, or individuals use the aforementioned content, they must clearly indicate the original source and origin of the work and assume legal responsibility on their own.